Astronomers have finally glimpsed the blackness of a black hole. By stringing together a global network of radio telescopes, they have for the first time produced a picture of an event horizon — a black hole’s perilous edge — against a backdrop of swirling light.
“We have seen the gates of hell at the end of space and time,” said astrophysicist Heino Falcke of Radboud University in Nijmegen, the Netherlands, at a press conference in Brussels. “What you’re looking at is a ring of fire created by the deformation of space-time. Light goes around, and looks like a circle.”
The first image of a black hole: A three minute guide
The images — of a glowing, ring-like structure — show the supermassive black hole at the centre of the galaxy M87, which is around 16 megaparsecs (55 million light years) away and 6.5 billion times the mass of the Sun. They reveal, in greater detail than ever before, the event horizon — the surface beyond which gravity is so strong that nothing that crosses it, even light, can ever climb back out.
The highly anticipated results, comparable to recognizing a doughnut on the Moon’s surface, were unveiled today by the Event Horizon Telescope (EHT) collaboration in seven simultaneous press conferences on four continents. The findings were also published in a suite of papers1,2,3,4,5 in Astrophysical Journal Letters on 10 April.
The image is a “tremendous accomplishment”, says astrophysicist Roger Blandford at Stanford University in California, who was not involved with the work. “When I was a student, I never dreamt that anything like this would be possible,” he says. “It is yet another confirmation of general relativity as the correct theory of strong gravity.”
“I was so delighted,” says Andrea Ghez, an astronomer at the University of California, Los Angeles. The images provide “clear evidence” of a ‘photon ring’ around a black hole, she says.
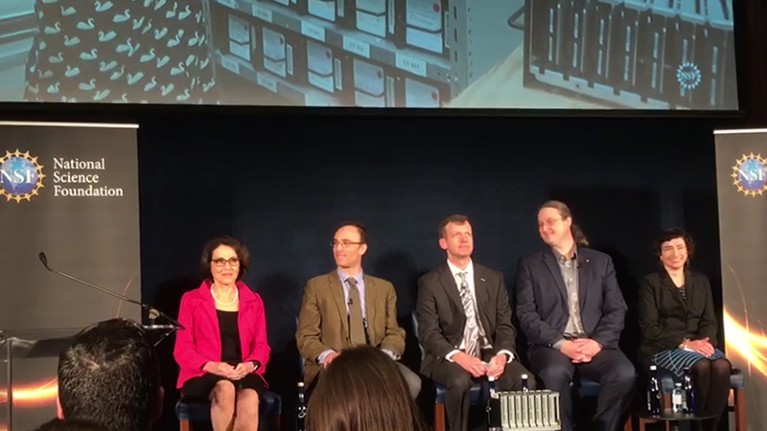
Six press conferences around the world revealed the black-hole images.
Black-hole predictions
Nearly a century ago, physicists first deduced that black holes should exist from Albert Einstein’s general theory of relativity, but most of the evidence so far has been indirect. The EHT has now made a new, spectacular confirmation of those predictions.
How scientists reacted to the first-ever image of a black hole
The team observed two supermassive black holes — M87’s and Sagittarius A*, the void at the Milky Way’s centre — over five nights in April 2017. They mustered enough resolution to capture the distant objects by linking up eight radio observatories across the globe — from Hawaii to the South Pole — and each collected more data than the Large Hadron Collider does in a year (see ‘Global effort’). The data set is likely to be the largest ever collected by a science experiment, and it took two years of work to produce the pictures.
After combining the observatories’ data, the team started analysis in mid-2018. They quickly realized that they could get a first, clean picture from M87. “We focused all our attention on M87 when we saw our first results because we saw this is going to be awesome,” says Falcke.
At the Brussels press conference, astrophysicist and collaboration member Monika Moscibrodzka, also at Radboud, said that the measurements so far are not precise enough to measure how fast the M87 hole spins — a crucial feature for a black hole. But it indicates the direction in which it’s spinning, which is clockwise in the sky, she said. Further studies could also help researchers understand how the black hole produces its gigantic jets.
The teams will also now turn their attention to the Sagittarius A* data. Because Sagittarius A* is nearly 1,000 times smaller than the M87 black hole, matter orbited it many times during each observing session, producing a rapidly changing signal rather than a steady one, says Luciano Rezzolla, a theoretical astrophysicist at the Goethe University Frankfurt in Germany and a member of the EHT team. That makes the data more complicated to interpret, but also potentially richer in information.
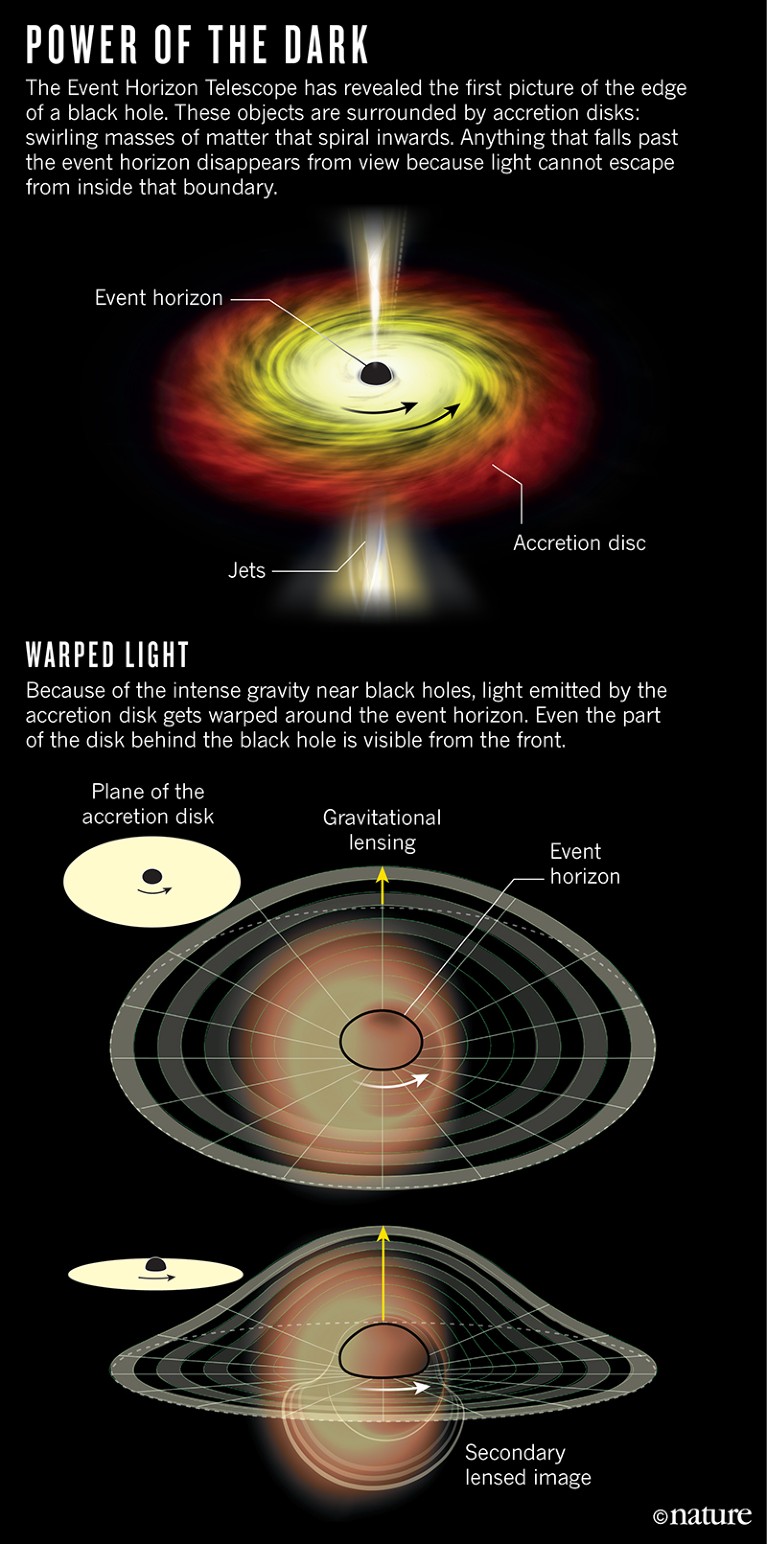
NIK SPENCER/Nature; Avery Broderick/University of Waterloo (IMAGES bottom)
Event horizons are the defining feature of black holes. To a nearby observer, an event horizon should appear as a spherical surface shrouding its interiors from view. Because light can cross the surface only one way — inwards — the globe should look completely black (see ‘Power of the dark’).
A black hole’s event horizon should appear five times larger than it is, because the hole warps the surrounding space and bends the paths of light. The effect, discovered by physicist James Bardeen at the University of Washington in Seattle in 1973, is similar to the way that a spoon looks larger when dipped in a glass of water. Moreover, Bardeen showed that the black hole would cast an even larger ‘shadow’. This is because within a certain distance of the event horizon, most light rays bend so much that they effectively orbit the black hole.
Earth-sized telescope
To actually resolve details on the scale of the event horizon, radio astronomers calculated that they would need a telescope the size of Earth (a telescope’s resolution is also proportional to its size). Fortunately, a technique called interferometry could help. It involves multiple telescopes, located far apart from one another and pointed at the same object simultaneously. Effectively, the telescopes work as if they were shards of one big dish.
How to hunt for a black hole with a telescope the size of Earth
Various teams around the world refined their techniques, and retrofitted some major observatories so that they could add them to a network. In particular, a group led by Shep Doeleman, now at Harvard University in Cambridge, Massachusetts, adapted the 10-metre South Pole Telescope and the US$1.4-billion Atacama Large Millimeter/submillimeter Array (ALMA) in Chile to do the work.
In 2014, Falcke, Doeleman and groups from around the world joined forces to form the EHT collaboration. They did their first Earth-spanning observation campaign in 2017. They observed Sagittarius A* and M87 during a two-week window in April when the locations of the observatories are most likely to get good weather simultaneously.
The raw data, which ran into petabytes, were collected on hard disks and travelled by air, sea and land to be compiled at the Max Planck Institute for Radio Astronomy in Bonn, Germany and the Massachusetts Institute of Technology’s Haystack Observatory in Westford.
Last year, while the data were still being processed, Falcke told Nature that he expected the experiment to gather a wealth of information about the structure of the black holes, but not yet a pretty picture. At best, it would resemble “an ugly peanut”, he said. “Or maybe, the first image will be just a few blots. It may not even resemble a peanut.”
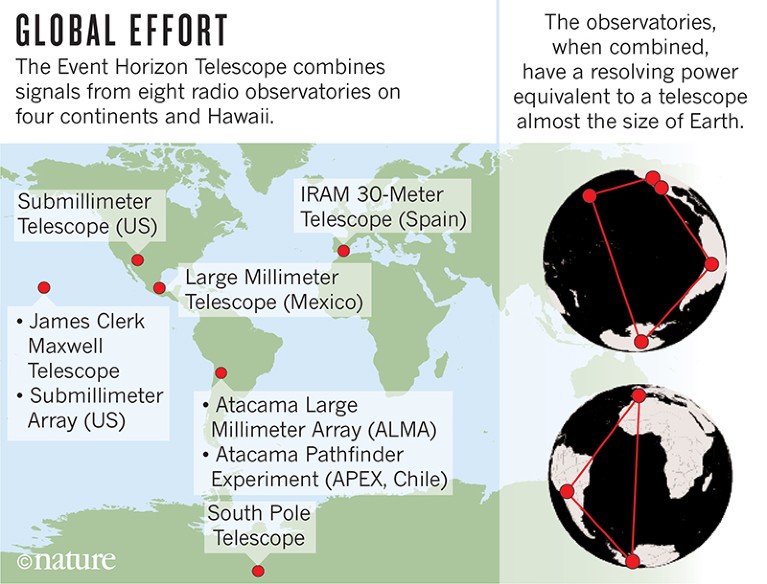
The EHT ran another observing campaign in 2018 — the analysis of those data is still in the works — but cancelled a planned observing campaign this year because of security issues near one of its most important sites, the 50-metre Large Millimeter Telescope (LMT) in Puebla, Mexico. They plan to continue to do observations once a year starting in 2020.
The collaboration is now looking for funding to establish a foothold in Africa, which would fill in a major gap in the network. The plan is to relocate a 15-metre dish — a decommissioned Swedish telescope — from Chile to the Gamsberg Table Mountain in Namibia. For now, the network has already secured two major additions: a dish in Greenland and an array in the French Alps.
An expanded EHT network could provide detail on what happens inside the voids — “how the world behaves inside black holes, and if it is as we expected it to be”, says David Sánchez Argüelles, a physicist at the LMT.
“It was a great sense of relief to see this, but also surprise,” says Doeleman of the results. “You know what I was really expecting to see? A blob. To see this ring is probably the best outcome that we could have had.”